肝线粒体功能障碍在非酒精性脂肪性肝病发病中的作用机制
DOI: 10.12449/JCH240124
利益冲突声明:本文不存在任何利益冲突。
作者贡献声明:杨冰清负责参考文献查阅及撰写论文;尹静亚参与论文修改;王琦负责综述构架制订,论文审校及最后定稿。
The mechanism of action of mitochondrial dysfunction in the development of non-alcoholic fatty liver disease
-
摘要: 非酒精性脂肪性肝病(NAFLD)逐渐成为影响人类肝脏健康的主要原因,其发生发展涉及多方面因素。线粒体作为细胞的“能量工厂”,对维持机体正常生理功能起着重要作用。研究表明,肝线粒体功能障碍促进NAFLD的发生发展。本文简要介绍了肝线粒体的基本特征和生理功能研究的新进展,综述了近年来线粒体功能障碍与肥胖、单纯性脂肪肝和非酒精性脂肪性肝炎关系研究方面的新成果,旨在为靶向线粒体治疗NAFLD提供研究思路。Abstract: Nonalcoholic fatty liver disease (NAFLD) has gradually become the main reason affecting human liver health, and many factors are involved in the development and progression of NAFLD. Mitochondria, as the “energy factory” of cells, plays an important role in maintaining normal physiological functions. Studies have shown that hepatic mitochondrial dysfunction promotes the development and progression of NAFLD. This article briefly introduces the latest research advances in the basic characteristics and physiological function of liver mitochondria and reviews new research findings in the association of mitochondrial dysfunction with obesity, simple fatty liver disease, and nonalcoholic steatohepatitis, in order to provide new ideas for the research on targeted mitochondrial therapy for NAFLD.
-
[1] TARGHER G, COREY KE, BYRNE CD, et al. The complex link between NAFLD and type 2 diabetes mellitus-mechanisms and treatments[J]. Nat Rev Gastroenterol Hepatol, 2021, 18( 9): 599- 612. DOI: 10.1038/s41575-021-00448-y. [2] PAFILI K, RODEN M. Nonalcoholic fatty liver disease(NAFLD) from pathogenesis to treatment concepts in humans[J]. Mol Metab, 2021, 50: 101122. DOI: 10.1016/j.molmet.2020.101122. [3] RIAZI K, AZHARI H, CHARETTE JH, et al. The prevalence and incidence of NAFLD worldwide: A systematic review and meta-analysis[J]. Lancet Gastroenterol Hepatol, 2022, 7( 9): 851- 861. DOI: 10.1016/S2468-1253(22)00165-0. [4] NOUREDDIN M, SANYAL AJ. Pathogenesis of NASH: The impact of multiple pathways[J]. Curr Hepatol Rep, 2018, 17( 4): 350- 360. DOI: 10.1007/s11901-018-0425-7. [5] MANSOURI A, GATTOLLIAT CH, ASSELAH T. Mitochondrial dysfunction and signaling in chronic liver diseases[J]. Gastroenterology, 2018, 155( 3): 629- 647. DOI: 10.1053/j.gastro.2018.06.083. [6] CIAULA AD, PASSARELLA S, SHANMUGAM H, et al. Nonalcoholic fatty liver disease(NAFLD). Mitochondria as players and targets of therapies?[J]. Int J Mol Sci, 2021, 22( 10): 5375. DOI: 10.3390/ijms22105375. [7] XIAO WC, AN W. Role of mitochondrial injury in the development and progression of nonalcoholic fatty liver disease[J]. J Clin Hepatol, 2021, 37( 7): 1515- 1521. DOI: 10.3969/j.issn.1001-5256.2021.07.005.肖卫纯, 安威. 线粒体损伤在非酒精性脂肪性肝病发生发展中的作用[J]. 临床肝胆病杂志, 2021, 37( 7): 1515- 1521. DOI: 10.3969/j.issn.1001-5256.2021.07.005. [8] TSVETKOV P, COY S, PETROVA B, et al. Copper induces cell death by targeting lipoylated TCA cycle proteins[J]. Science, 2022, 375( 6586): 1254- 1261. DOI: 10.1126/science.abf0529. [9] NI HM, WILLIAMS JA, DING WX. Mitochondrial dynamics and mitochondrial quality control[J]. Redox Biol, 2015, 4: 6- 13. DOI: 10.1016/j.redox.2014.11.006. [10] YOULE RJ, van DER BLIEK AM. Mitochondrial fission, fusion, and stress[J]. Science, 2012, 337( 6098): 1062- 1065. DOI: 10.1126/science.1219855. [11] CHOWANADISAI W, BAUERLY KA, TCHAPARIAN E, et al. Pyrroloquinoline quinone stimulates mitochondrial biogenesis through cAMP response element-binding protein phosphorylation and increased PGC-1alpha expression[J]. J Biol Chem, 2010, 285( 1): 142- 152. DOI: 10.1074/jbc.M109.030130. [12] KOLIAKI C, RODEN M. Alterations of mitochondrial function and insulin sensitivity in human obesity and diabetes mellitus[J]. Annu Rev Nutr, 2016, 36: 337- 367. DOI: 10.1146/annurev-nutr-071715-050656. [13] XIAN HX, LIOU YC. Functions of outer mitochondrial membrane proteins: Mediating the crosstalk between mitochondrial dynamics and mitophagy[J]. Cell Death Differ, 2021, 28( 3): 827- 842. DOI: 10.1038/s41418-020-00657-z. [14] KOLIAKI C, SZENDROEDI J, KAUL K, et al. Adaptation of hepatic mitochondrial function in humans with non-alcoholic fatty liver is lost in steatohepatitis[J]. Cell Metab, 2015, 21( 5): 739- 746. DOI: 10.1016/j.cmet.2015.04.004. [15] POUSSIN C, IBBERSON M, HALL D, et al. Oxidative phosphorylation flexibility in the liver of mice resistant to high-fat diet-induced hepatic steatosis[J]. Diabetes, 2011, 60( 9): 2216- 2224. DOI: 10.2337/db11-0338. [16] PETERSEN KF, BEFROY DE, DUFOUR S, et al. Assessment of hepatic mitochondrial oxidation and pyruvate cycling in NAFLD by(13)C magnetic resonance spectroscopy[J]. Cell Metab, 2016, 24( 1): 167- 171. DOI: 10.1016/j.cmet.2016.06.005. [17] SUNNY NE, PARKS EJ, BROWNING JD, et al. Excessive hepatic mitochondrial TCA cycle and gluconeogenesis in humans with nonalcoholic fatty liver disease[J]. Cell Metab, 2011, 14( 6): 804- 810. DOI: 10.1016/j.cmet.2011.11.004. [18] PEDERSEN JS, RYGG MO, CHRØIS K, et al. Influence of NAFLD and bariatric surgery on hepatic and adipose tissue mitochondrial biogenesis and respiration[J]. Nat Commun, 2022, 13( 1): 2931. DOI: 10.1038/s41467-022-30629-5. [19] SATAPATI S, KUCEJOVA B, DUARTE JAG, et al. Mitochondrial metabolism mediates oxidative stress and inflammation in fatty liver[J]. J Clin Invest, 2016, 126( 4): 1605. DOI: 10.1172/JCI86695. [20] SHAMI GJ, CHENG D, VERHAEGH P, et al. Three-dimensional ultrastructure of giant mitochondria in human non-alcoholic fatty liver disease[J]. Sci Rep, 2021, 11( 1): 3319. DOI: 10.1038/s41598-021-82884-z. [21] XU ZX, LUO SZ, XU MY. Mechanism of mitochondrial dysfunction in the development of nonalcoholic fatty liver disease[J]. J Clin Hepatol, 2020, 36( 10): 2353- 2355. DOI: 10.3969/j.issn.1001-5256.2020.10.042.徐梓馨, 罗声政, 徐铭益. 线粒体功能异常在非酒精性脂肪性肝病发生中的机制[J]. 临床肝胆病杂志, 2020, 36( 10): 2353- 2355. DOI: 10.3969/j.issn.1001-5256.2020.10.042. [22] LI YF, XU JY, LU YT, et al. DRAK2 aggravates nonalcoholic fatty liver disease progression through SRSF6-associated RNA alternative splicing[J]. Cell Metab, 2021, 33( 10): 2004- 2020. DOI: 10.1016/j.cmet.2021.09.008. [23] CHAVIN KD, YANG S, LIN HZ, et al. Obesity induces expression of uncoupling protein-2 in hepatocytes and promotes liver ATP depletion[J]. J Biol Chem, 1999, 274( 9): 5692- 5700. DOI: 10.1074/jbc.274.9.5692. [24] CORTEZ-PINTO H, CHATHAM J, CHACKO VP, et al. Alterations in liver ATP homeostasis in human nonalcoholic steatohepatitis: A pilot study[J]. JAMA, 1999, 282( 17): 1659- 1664. DOI: 10.1001/jama.282.17.1659. [25] MORRIS EM, RECTOR RS, THYFAULT JP, et al. Mitochondria and redox signaling in steatohepatitis[J]. Antioxid Redox Signal, 2011, 15( 2): 485- 504. DOI: 10.1089/ars.2010.3795. [26] BEGRICHE K, IGOUDJIL A, PESSAYRE D, et al. Mitochondrial dysfunction in NASH: Causes, consequences and possible means to prevent it[J]. Mitochondrion, 2006, 6( 1): 1- 28. DOI: 10.1016/j.mito.2005.10.004. [27] XU JS, CHEN SY, WANG W, et al. Hepatic CDP-diacylglycerol synthase 2 deficiency causes mitochondrial dysfunction and promotes rapid progression of NASH and fibrosis[J]. Sci Bull, 2022, 67( 3): 299- 314. DOI: 10.1016/j.scib.2021.10.014. [28] MIYAO M, KAWAI C, KOTANI H, et al. Mitochondrial fission in hepatocytes as a potential therapeutic target for nonalcoholic steatohepatitis[J]. Hepatol Res, 2022, 52( 12): 1020- 1033. DOI: 10.1111/hepr.13832. [29] MONTEIRO JP, OLIVEIRA PJ, JURADO AS. Mitochondrial membrane lipid remodeling in pathophysiology: A new target for diet and therapeutic interventions[J]. Prog Lipid Res, 2013, 52( 4): 513- 528. DOI: 10.1016/j.plipres.2013.06.002. [30] MAGKOS F, SU X, BRADLEY D, et al. Intrahepatic diacylglycerol content is associated with hepatic insulin resistance in obese subjects[J]. Gastroenterology, 2012, 142( 7): 1444- 1446. DOI: 10.1053/j.gastro.2012.03.003. [31] APOSTOLOPOULOU M, GORDILLO R, KOLIAKI C, et al. Specific hepatic sphingolipids relate to insulin resistance, oxidative stress, and inflammation in nonalcoholic steatohepatitis[J]. Diabetes Care, 2018, 41( 6): 1235- 1243. DOI: 10.2337/dc17-1318. [32] OOI GJ, MEIKLE PJ, HUYNH K, et al. Hepatic lipidomic remodeling in severe obesity manifests with steatosis and does not evolve with non-alcoholic steatohepatitis[J]. J Hepatol, 2021, 75( 3): 524- 535. DOI: 10.1016/j.jhep.2021.04.013. [33] GAO RX, LI Y, XU ZM, et al. Mitochondrial pyruvate carrier 1 regulates fatty acid synthase lactylation and mediates treatment of nonalcoholic fatty liver disease[J]. Hepatology, 2023, 78( 6): 1800- 1815. DOI: 10.1097/HEP.0000000000000279. [34] LI Y, XIU WJ, XU JW, et al. Increased CHCHD2 expression promotes liver fibrosis in nonalcoholic steatohepatitis via Notch/osteopontin signaling[J]. JCI Insight, 2022, 7( 23): e162402. DOI: 10.1172/jci.insight.162402. [35] HE YH, ZHU MX, LIU YY, et al. Research progress of Curcumin and Resveratrol in the treatment of non-alcoholic fatty liver disease[J]. Chin Med Herald, 2023, 20( 1): 57- 60. DOI: 10.20047/j.issn1673-7210.2023.01.12.贺玉慧, 朱铭星, 刘跃洋, 等. 姜黄素与白藜芦醇治疗非酒精性脂肪性肝病的机制研究进展[J]. 中国医药导报, 2023, 20( 1): 57- 60. DOI: 10.20047/j.issn1673-7210.2023.01.12. [36] GEORGIEV A, GRANATA C, RODEN M. The role of mitochondria in the pathophysiology and treatment of common metabolic diseases in humans[J]. Am J Physiol Cell Physiol, 2022, 322( 6): C1248- C1259. DOI: 10.1152/ajpcell.00035.2022. [37] GRANATA C, JAMNICK NA, BISHOP DJ. Training-induced changes in mitochondrial content and respiratory function in human skeletal muscle[J]. Sports Med, 2018, 48( 8): 1809- 1828. DOI: 10.1007/s40279-018-0936-y. [38] THIETART S, RAUTOU PE. Extracellular vesicles as biomarkers in liver diseases: A clinician’s point of view[J]. J Hepatol, 2020, 73( 6): 1507- 1525. DOI: 10.1016/j.jhep.2020.07.014. [39] AMIR DACHE Z AL, OTANDAULT A, TANOS R, et al. Blood contains circulating cell-free respiratory competent mitochondria[J]. FASEB J, 2020, 34( 3): 3616- 3630. DOI: 10.1096/fj.201901917RR. -
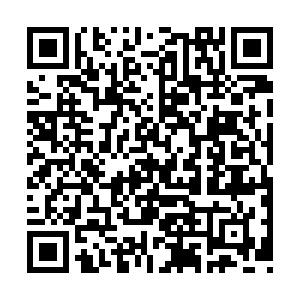
计量
- 文章访问数: 741
- HTML全文浏览量: 406
- PDF下载量: 159
- 被引次数: 0