线粒体障碍在非酒精性脂肪性肝炎中的作用机制
DOI: 10.3969/j.issn.1001-5256.2021.08.045
利益冲突声明:所有作者均声明不存在利益冲突。
作者贡献声明:蔡恬莹负责课题设计,资料分析和撰写论文;黄美州、陈浩、钱保林参与收集数据,修改论文;付文广负责拟定写作思路,指导撰写文章并最后定稿。
Mechanism of action of mitochondrial disorders in nonalcoholic steatohepatitis
-
摘要: 非酒精性脂肪性肝炎(NASH)是一种以肝脂肪变性、肝细胞炎症和肝纤维化为主要特征的常见慢性肝病。研究表明,线粒体内脂质代谢异常和活性氧生成、线粒体呼吸链受损、线粒体破裂以及线粒体自噬异常等线粒体障碍在NASH的发生、发展及转归过程中发挥重要作用。以NASH中肝脂肪代谢异常、肝炎发生和肝纤维化为主要线索,总结线粒体结构破坏、功能障碍以及线粒体自噬受损在NASH中的作用机制,以寻找相应靶点指导对NASH的治疗。Abstract: Nonalcoholic steatohepatitis (NASH) is a common chronic liver disease characterized by hepatic steatosis, hepatocyte inflammation, and liver fibrosis. Studies have shown that mitochondrial disorders, such as abnormal lipid metabolism, reactive oxygen generation, damaged mitochondrial respiratory chain, mitochondrial rupture, and abnormal mitochondrial autophagy, play an important role in the development, progression, and prognosis of NASH. With the main clues of abnormal lipid metabolism, hepatitis, and liver fibrosis in NASH, this article reviews the mechanism of action of mitochondrial structural destruction, mitochondrial dysfunction, and impaired mitochondrial autophagy in NASH, so as to find corresponding targets for the treatment of NASH.
-
Key words:
- Non-Alcoholic Fatty Liver Disease /
- Mitochondria /
- Autophagy
-
[1] ANJANI K, LHOMME M, SOKOLOVSKA N, et al. Circulating phospholipid profiling identifies portal contribution to NASH signature in obesity[J]. J Hepatol, 2015, 62(4): 905-912. DOI: 10.1016/j.jhep.2014.11.002. [2] KNEBEL B, HARTWIG S, HAAS J, et al. Peroxisomes compensate hepatic lipid overflow in mice with fatty liver[J]. Biochim Biophys Acta, 2015, 1851(7): 965-976. DOI: 10.1016/j.bbalip.2015.03.003. [3] GAMBINO R, MUSSO G, CASSADER M. Redox balance in the pathogenesis of nonalcoholic fatty liver disease: Mechanisms and therapeutic opportunities[J]. Antioxid Redox Signal, 2011, 15(5): 1325-1365. DOI: 10.1089/ars.2009.3058. [4] ANDERSON A, CAMPO A, FULTON E, et al. 7-Ketocholesterol in disease and aging[J]. Redox Biol, 2020, 29: 101380. DOI: 10.1016/j.redox.2019.101380. [5] SUN X, SEIDMAN JS, ZHAO P, et al. Neutralization of oxidized phospholipids ameliorates non-alcoholic steatohepatitis[J]. Cell Metab, 2020, 31(1): 189-206. e8. DOI: 10.1016/j.cmet.2019.10.014. [6] PIROLA CJ, GARAYCOECHEA M, FLICHMAN D, et al. Liver mitochondrial DNA damage and genetic variability of Cytochrome b - a key component of the respirasome - drive the severity of fatty liver disease[J]. J Intern Med, 2021, 289(1): 84-96. DOI: 10.1111/joim.13147. [7] MALIK AN, SIMÖES I, ROSA HS, et al. A diet induced maladaptive increase in hepatic mitochondrial DNA precedes OXPHOS defects and may contribute to non-alcoholic fatty liver disease[J]. Cells, 2019, 8(10): 1222. DOI: 10.3390/cells8101222. [8] ITEM F, WUEEST S, LEMOS V, et al. Fas cell surface death receptor controls hepatic lipid metabolism by regulating mitochondrial function[J]. Nat Commun, 2017, 8(1): 480. DOI: 10.1038/s41467-017-00566-9. [9] EL-DERANY MO, EL-DEMERDASH E. Pyrvinium pamoate attenuates non-alcoholic steatohepatitis: Insight on hedgehog/Gli and Wnt/β-catenin signaling crosstalk[J]. Biochem Pharmacol, 2020, 177: 113942. DOI: 10.1016/j.bcp.2020.113942. [10] WANG L, LIU X, NIE J, et al. ALCAT1 controls mitochondrial etiology of fatty liver diseases, linking defective mitophagy to steatosis[J]. Hepatology, 2015, 61(2): 486-496. DOI: 10.1002/hep.27420. [11] PARK HS, SONG JW, PARK JH, et al. TXNIP/VDUP1 attenuates steatohepatitis via autophagy and fatty acid oxidation[J]. Autophagy, 2020. DOI: 10.1080/15548627.2020.1834711.[Onlineaheadofprint] [12] SCHRÖDER T, KUCHARCZYK D, BÄR F, et al. Mitochondrial gene polymorphisms alter hepatic cellular energy metabolism and aggravate diet-induced non-alcoholic steatohepatitis[J]. Mol Metab, 2016, 5(4): 283-295. DOI: 10.1016/j.molmet.2016.01.010. [13] HOYT LR, RANDALL MJ, ATHER JL, et al. Mitochondrial ROS induced by chronic ethanol exposure promote hyper-activation of the NLRP3 inflammasome[J]. Redox Biol, 2017, 12: 883-896. DOI: 10.1016/j.redox.2017.04.020. [14] ZOROV DB, FILBURN CR, KLOTZ LO, et al. Reactive oxygen species (ROS)-induced ROS release: A new phenomenon accompanying induction of the mitochondrial permeability transition in cardiac myocytes[J]. J Exp Med, 2000, 192(7): 1001-1014. DOI: 10.1084/jem.192.7.1001. [15] ANTONIEL M, JONES K, ANTONUCCI S, et al. The unique histidine in OSCP subunit of F-ATP synthase mediates inhibition of the permeability transition pore by acidic pH[J]. EMBO Rep, 2018, 19(2): 257-268. DOI: 10.15252/embr.201744705. [16] WEST AP, SHADEL GS. Mitochondrial DNA in innate immune responses and inflammatory pathology[J]. Nat Rev Immunol, 2017, 17(6): 363-375. DOI: 10.1038/nri.2017.21. [17] ZHONG Z, UMEMURA A, SANCHEZ-LOPEZ E, et al. NF-κB restricts inflammasome activation via elimination of damaged mitochondria[J]. Cell, 2016, 164(5): 896-910. DOI: 10.1016/j.cell.2015.12.057. [18] GUHA P, TYAGI R, CHOWDHURY S, et al. IPMK mediates activation of ULK signaling and transcriptional regulation of autophagy linked to liver inflammation and regeneration[J]. Cell Rep, 2019, 26(10): 2692-2703. e7. DOI: 10.1016/j.celrep.2019.02.013. [19] DE GREGORIO E, COLELL A, MORALES A, et al. Relevance of SIRT1-NF-κB axis as therapeutic target to ameliorate inflammation in liver disease[J]. Int J Mol Sci, 2020, 21(11): 3858. DOI: 10.3390/ijms21113858. [20] WIDJAJA AA, SINGH BK, ADAMI E, et al. Inhibiting interleukin 11 signaling reduces hepatocyte death and liver fibrosis, inflammation, and steatosis in mouse models of nonalcoholic steatohepatitis[J]. Gastroenterology, 2019, 157(3): 777-792. e14. DOI: 10.1053/j.gastro.2019.05.002. [21] DAS N, MANDALA A, NAAZ S, et al. Melatonin protects against lipid-induced mitochondrial dysfunction in hepatocytes and inhibits stellate cell activation during hepatic fibrosis in mice[J]. J Pineal Res, 2017, 62(4): e12404. DOI: 10.1111/jpi.12404. [22] FRANGOGIANNIS N. Transforming growth factor-β in tissue fibrosis[J]. J Exp Med, 2020, 217(3): e20190103. DOI: 10.1084/jem.20190103. [23] CHERESH P, KIM SJ, TULASIRAM S, et al. Oxidative stress and pulmonary fibrosis[J]. Biochim Biophys Acta, 2013, 1832(7): 1028-1040. DOI: 10.1016/j.bbadis.2012.11.021. [24] OKINA Y, SATO-MATSUBARA M, MATSUBARA T, et al. TGF-β1-driven reduction of cytoglobin leads to oxidative DNA damage in stellate cells during non-alcoholic steatohepatitis[J]. J Hepatol, 2020, 73(4): 882-895. DOI: 10.1016/j.jhep.2020.03.051. [25] AN P, WEI LL, ZHAO S, et al. Hepatocyte mitochondria-derived danger signals directly activate hepatic stellate cells and drive progression of liver fibrosis[J]. Nat Commun, 2020, 11(1): 2362. DOI: 10.1038/s41467-020-16092-0. [26] MARCELIN G, da CUNHA C, GAMBLIN C, et al. Autophagy inhibition blunts PDGFRA adipose progenitors' cell-autonomous fibrogenic response to high-fat diet[J]. Autophagy, 2020, 16(12): 2156-2166. DOI: 10.1080/15548627.2020.1717129. [27] McCOMMIS KS, FINCK BN. Treating hepatic steatosis and fibrosis by modulating mitochondrial pyruvate metabolism[J]. Cell Mol Gastroenterol Hepatol, 2019, 7(2): 275-284. DOI: 10.1016/j.jcmgh.2018.09.017. [28] LIU J, LI D, ZHANG T, et al. SIRT3 protects hepatocytes from oxidative injury by enhancing ROS scavenging and mitochondrial integrity[J]. Cell Death Dis, 2017, 8(10): e3158. DOI: 10.1038/cddis.2017.564. [29] MANSOURI A, GATTOLLIAT CH, ASSELAH T. Mitochondrial dysfunction and signaling in chronic liver diseases[J]. Gastroenterology, 2018, 155(3): 629-647. DOI: 10.1053/j.gastro.2018.06.083. [30] ZENG X, YANG J, HU O, et al. Dihydromyricetin ameliorates nonalcoholic fatty liver disease by improving mitochondrial respiratory capacity and redox homeostasis through modulation of SIRT3 signaling[J]. Antioxid Redox Signal, 2019, 30(2): 163-183. DOI: 10.1089/ars.2017.7172. [31] SINHA K, DAS J, PAL PB, et al. Oxidative stress: The mitochondria-dependent and mitochondria-independent pathways of apoptosis[J]. Arch Toxicol, 2013, 87(7): 1157-1180. DOI: 10.1007/s00204-013-1034-4. [32] TAIT SW, GREEN DR. Mitochondria and cell death: Outer membrane permeabilization and beyond[J]. Nat Rev Mol Cell Biol, 2010, 11(9): 621-632. DOI: 10.1038/nrm2952. [33] DU J, ZHANG X, HAN J, et al. Pro-inflammatory CXCR3 impairs mitochondrial function in experimental non-alcoholic steatohepatitis[J]. Theranostics, 2017, 7(17): 4192-4203. DOI: 10.7150/thno.21400. [34] ANDUEZA A, GARDE N, GARCÍA-GARZÓN A, et al. NADPH oxidase 5 promotes proliferation and fibrosis in human hepatic stellate cells[J]. Free Radic Biol Med, 2018, 126: 15-26. DOI: 10.1016/j.freeradbiomed.2018.07.013. [35] ZHAO Q, LIU J, DENG H, et al. Targeting mitochondria-located circRNA SCAR alleviates NASH via reducing mROS output[J]. Cell, 2020, 183(1): 76-93. e22. DOI: 10.1016/j.cell.2020.08.009. [36] LV F, LI N, KONG M, et al. CDKN2a/p16 antagonizes hepatic stellate cell activation and liver fibrosis by modulating ROS levels[J]. Front Cell Dev Biol, 2020, 8: 176. DOI: 10.3389/fcell.2020.00176. [37] WU X, POULSEN KL, SANZ-GARCIA C, et al. MLKL-dependent signaling regulates autophagic flux in a murine model of non-alcohol-associated fatty liver and steatohepatitis[J]. J Hepatol, 2020, 73(3): 616-627. DOI: 10.1016/j.jhep.2020.03.023 -
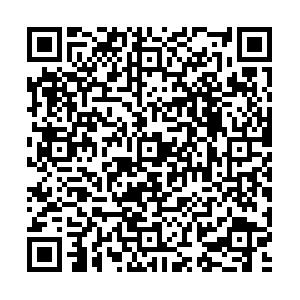
计量
- 文章访问数: 663
- HTML全文浏览量: 256
- PDF下载量: 90
- 被引次数: 0